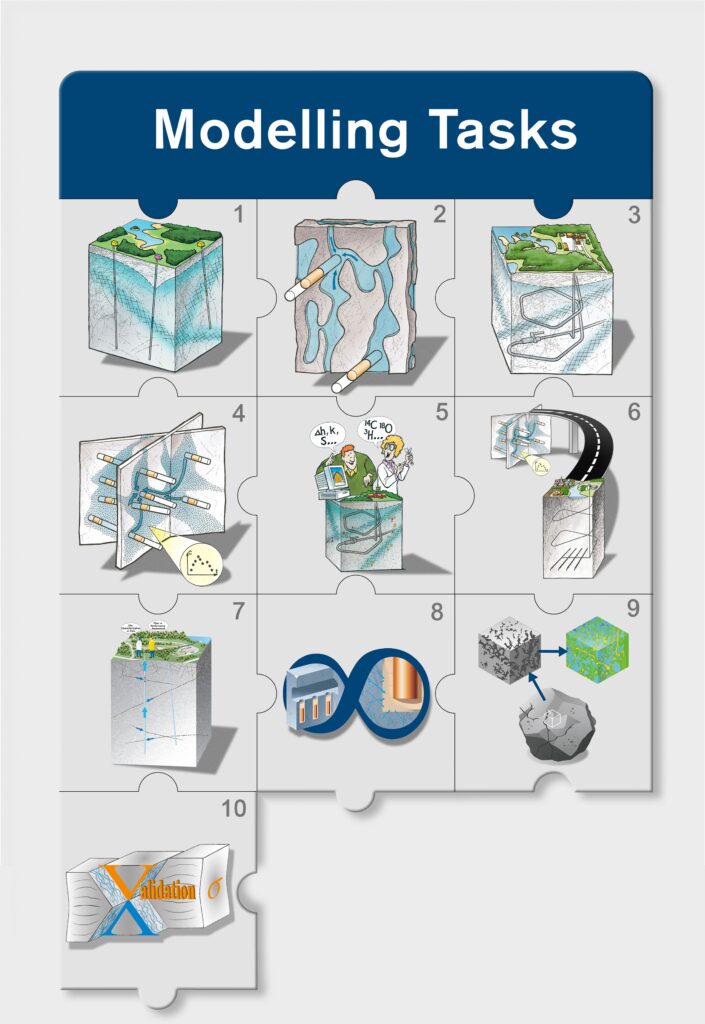
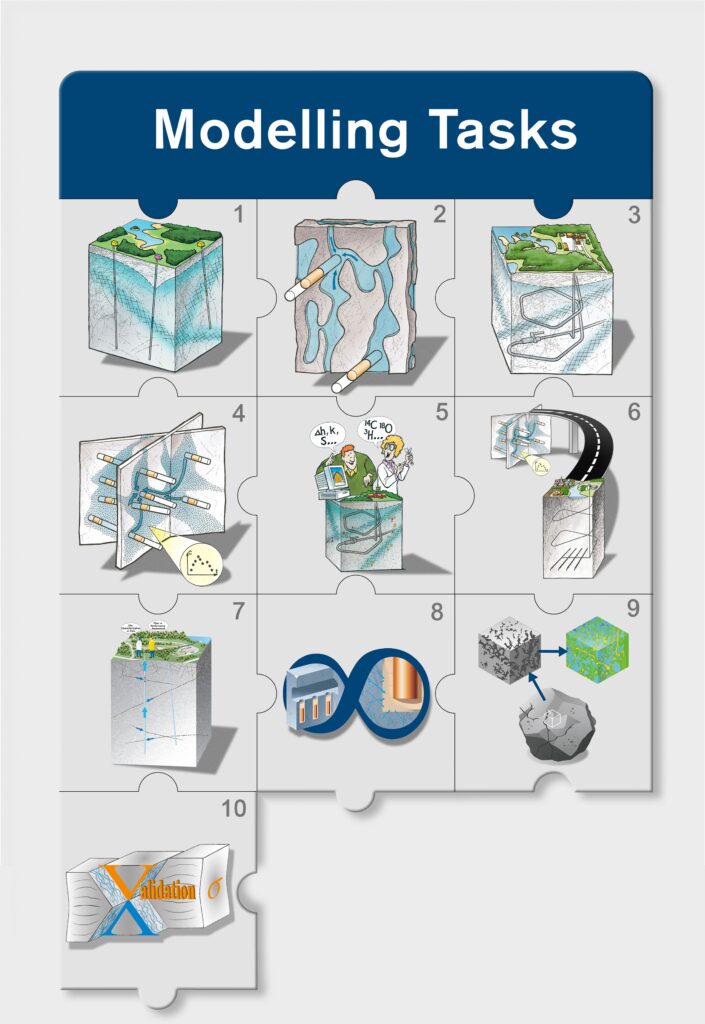
Task Force GWFTS modelling tasks
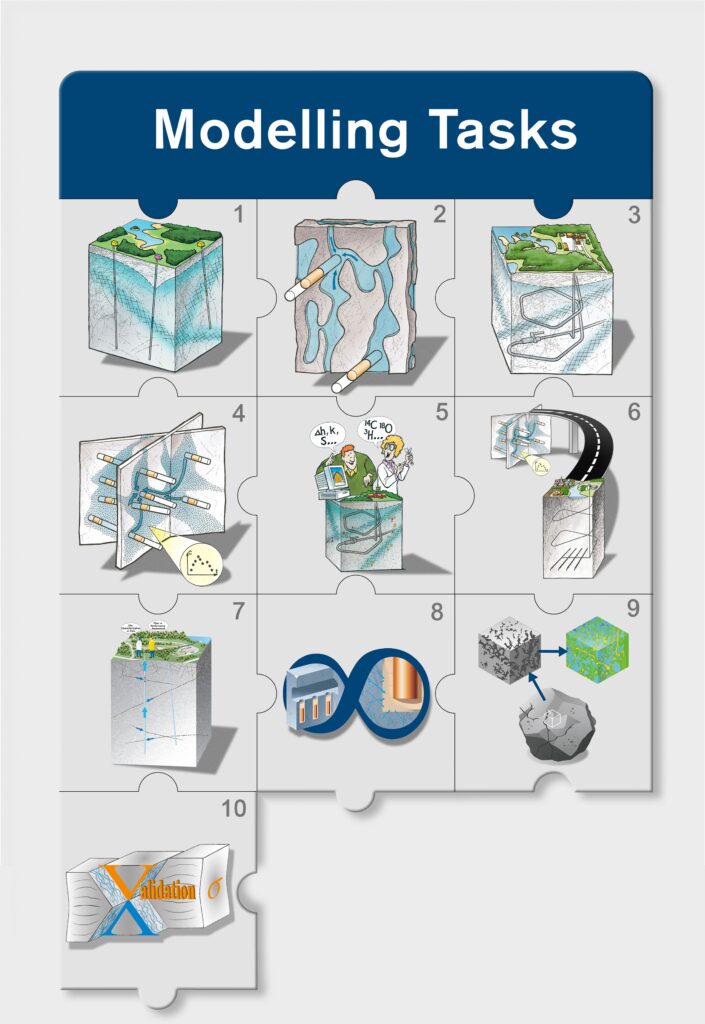
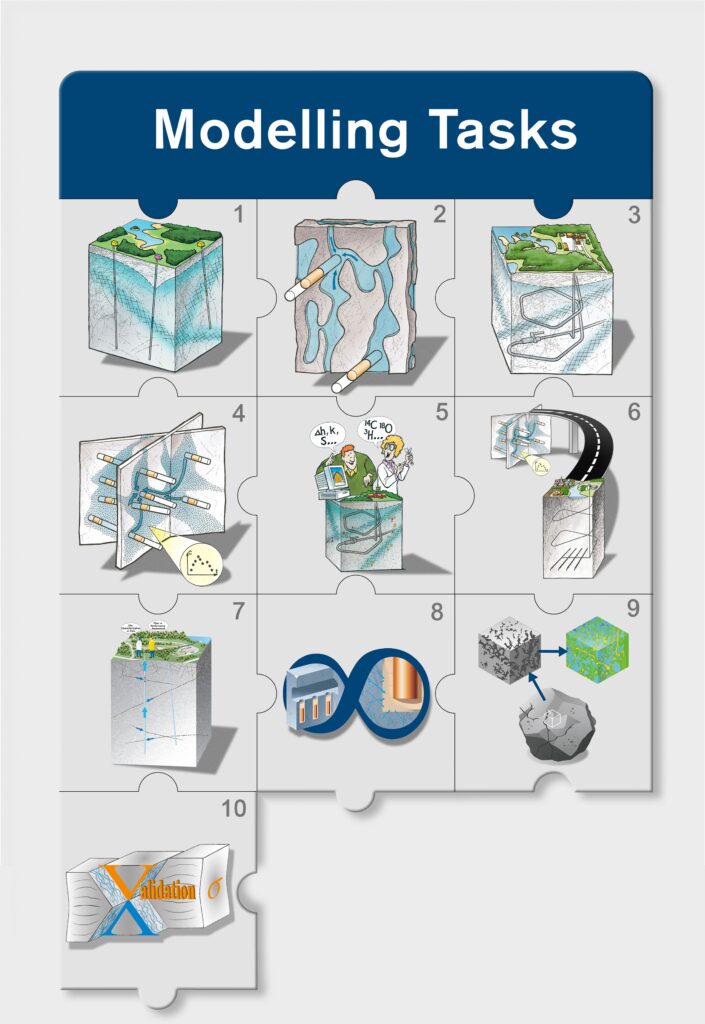
Task Force GWFTS modelling tasks
The work within the SKB Task Force GWFTS is performed within a framework of well-defined and focused Modelling Tasks. These are tied to experiments at Äspö HRL or another laboratory that can provide suitable data for modelling tasks.
Task 1 - The LPT2 experiments, evaluation modelling
Pumping, tracer and dilution tests on a site scale (1 km).
As the first task, a large scale field experiment was chosen. This consisted of a long term pumping test, dilution tests as well as a series of tracer tests. The modelling work performed during 1992-1994 has been evaluated by the Task Force group. The experiences are discussed from the modelling point of view, the Äspö data collection point of view and the site characterisation point of view.
International Cooperation Reports concerning Task 1
Status
This task is completed.
Task 2 - Äspö field tracer experiments, design modelling
Design calculations for a number of planned experiments at the Äspö site on a detailed scale (1-10m).
International Cooperation Reports concerning Task 2
Status
This task is completed.
Task 3 - The Äspö tunnel experiment, predictive/evaluation modelling
The hydraulic impact of the Äspö tunnel excavation, site scale (1 km).
The hydraulic impact of the Äspö Hard Rock Laboratory tunnel excavation was chosen as the third task.
The modelling work performed during 1994-1996 has been evaluated by the SKB Task Force group. The experiences are discussed from the modelling point of view, the Äspö data collection point of view and the site characterisation point of view.
Seven different groups modelled Task No 3 using different conceptual and numerical methodologies for simulating flow in fractured rocks. The modelling work has been reported within the Äspö ICR Series.
International Cooperation Reports concerning Task 3
Status
This task is completed.
Task 4 - Tracer Retention and Understanding Experiments - TRUE-1, predictive modelling
Radially converging tracer tests as well as dipole tests, detailed scale (1-10m).
Task 4 consists of modelling exercises in support of the TRUE tracer tests. Predictive modelling is also performed where the experimental results are not available beforehand.
International Cooperation Reports concerning Task 4
Status
Task completed.
Modelling Task | Definition/Scope |
4A | Modelling in support of the development of a descriptive structural model of the test site |
4B | Modelling in support of experimental design |
4C | Predictive modelling of the radially converging tracer tests in the feature. Comparison of model output with experimental results. |
4D | Predictive modelling of the dipole tests in the feature. Comparison of model output with experimental results. Evaluate what can be achieved with existing data set of TRUE-1 in terms of transport predictions for non-sorbing tracers. |
4E | Predictive modelling of the TRUE-1 tracer tests with sorbing tracers. Comparison of model output with experimental results. |
4F | Evaluation of what can be achieved with existing in-situ and laboratory data from the TRUE-1 site in terms of predicting transport of solutes subject to sorption. |
Task 5 - Integration of hydrogeology and hydrochemistry
A hydrogeological-hydrochemical model assessment exercise which specifically studies the impact of the Hard Rock Laboratory tunnel construction on the groundwater system at Äspö.
International Progress Reports concerning Task 5
Status
The task is completed.
Task 5 commenced in 1998 and was finalised in 2002. Participating modelling teams in the project represented ANDRA (France; three modelling teams – ANTEA, ITASCA, CEA), BMWi/BGR (Germany), ENRESA (Spain), JNC (Japan), CRIEPI (Japan), Posiva (Finland) and SKB (Sweden; two modelling teams – CFE and Intera (now GeoPoint)).
Experience from Task 5 has highlighted several important aspects for site investigations facilitating the possibilities for mathematically integrated modelling and consistency checks that should be taken into account for future repository performance assessments.
Equally important is that Task 5 has provided the opportunity to bring together two scientific disciplines which have traditionally tended to work in parallel rather in collaboration. This is an important first step. There is now a much stronger appreciation that the use of hydrogeochemistry can lead to an increased understanding of hydrogeology and vice-versa.
Task 6 - Performance Assessment Modelling Using Site Characterisation Data (PASC)
Solute transport is a key aspect of both Performance Assessment (PA) and repository Site Characterisation (SC). Task 6 seeks to provide a bridge between SC and PA approaches to solute transport in fractured rock and focuses on the 50 to 100m scale. Task 6 tries to bridge the gap between PA and SC models by applying both approaches for SC boundary conditions (i.e. for trace experiments), and also for PA boundary conditions.
The objectives of Task 6 are:
- Assess simplifications used in PA models
- Assess the constraining power of tracer (and flow) experiments for PA models
- Provide input for SC programs from a PA perspective
- Understand the site-specific flow and transport behaviour at scales using SC models.
Status
The task is completed
The contents of the model assignments within Task 6 are summarised in the table below.
Subtask | Contents |
6A | Model and reproduce selected True-1 tests with a PA model and/or a SC model to provide a common reference. |
6B | Model selected PA cases at the True-1 site with new PA relevant (long term/base case) boundary conditions and temporal scales. This task serves as means to understand the differences between the use of SC-type and PA-type models, and the influence of various assumptions made for PA calculations for extrapolation in time. |
6C | Develop semi-synthetic, fractured granite hydrostructural models. Two scales are supported (200 m block scale and 2000 m site-scale). The models are developed based on data from the Prototype Repository, True Block Scale, True-1, and Fracture Characterisation and Classification project (FCC). |
6D | This sub-task is similar to sub-task 6A, and is using the synthetic structural model in addition to a 50 to 100 m scale True-Block Scale tracer experiment. |
6E | This sub-task extends the sub-task 6D transport calculations to a reference set of PA time scales and boundary conditions. |
6F | Task 6F is a sensitivity study, which is proposed to address simple test cases, individual tasks to explore processes, and to test model functionality. |
Task 7 - Long-term pumping test at Olkilouto
The pumping test is performed at a scale of 100-1000 metres, using Posiva-flow logging (PFL) in open boreholes, and conventional pressure observations in open and packed-off boreholes.
Task 7 concerns modelling of a long-term pumping experiment at the Olkiluoto site in Finland. The task lies within a broader setting of site investigations, site characterisations, and ultimately safety assessment issues. The focus is especially on increased understanding of the major fracture zones behaviour as boundaries for bedrock compartments and the interaction between these “isolated” compartments and the major flow system. The experiences are supposed to provide a bridge between site characterisation and safety assessments.
Task 8 - Modelling of the Bentonite Rock Interaction Experiment, BRIE
Interactions between engineered and natural barriers
Task 8 was designed to model the Bentonite Rock Interaction Experiment (BRIE) i.e. the interactions between natural and engineered barriers. The task was performed together with another task force, i.e. the Engineered Barrier TF, and provided an increased insight of the interaction between bentonite and fractured rock e.g. at this interface for deposition holes (Finsterle et al., 2018, Finsterle 2019). More specifically, the objective of the task was to enhance the understanding and increase our ability to model:
Deposition boreholes in their tunnel environment
- Assessment of feasible deposition boreholes
- The hydraulic interaction between the rock and water unsaturated bentonite in a deposition borehole
- The effects of re-saturation on the flow system
- The understanding of post-resaturation flow and transport
Bentonite Rock Interaction Experiment (BRIE)
In order to obtain data for the modelling, the supporting field experiment, BRIE (Fransson et al. 2017), at Äspö Hard Rock Laboratory was carried out. In the experiment, two boreholes, both 30 cm in diameter, were filled with unsaturated bentonite and instrumented. Thus, these boreholes may be regarded as down-scaled deposition holes. One of these surrogate deposition holes was drilled in a part of the rock domain that is containing a range of water conducting fractures. The other hole was drilled in a part of the rock domain with very few and less water conductive fractures. Total stress, pore water pressure and relative humidity (RH) were measured in the bentonite. In the rock, pore water pressure and RH were measured close to the bore-holes. After suitable time, the holes were over-cored and the bentonite analyzed mainly regarding water content distribution. In the experiment, new technologies were required to:
- characterize the hydraulic conductivity of the rock for extremely low values
- characterize the dual porosity/dual permeability system
- monitor stress and water saturation in detail
- characterize the stress/pressure/permeability coupling
Modelling of BRIE – Task 8
The modelling task was to model the evolution in space and time of:
- The rock: the water pressure and water flow in the rock matrix and fractures around a deposition borehole.
- The bentonite: the water pressure, total pressure (swelling pressure), density and water content in a deposition borehole.
- The interface: Exchange of water over the interface between the bentonite and a sparsely fractured rock. The interface as a mutual boundary condition.
Task 8 proved to be an excellent learning exercise for both modelers normally dealing with fractured rocks and those focusing on bentonite, i.e., the interchange of information and knowledge between these communities was very fruitful (Finsterle et al. 2018). Also, the experimentalist made advancements dealing with this new challenge.
References
Finsterle S, Lanyon B, Åkesson M, Baxter S, Bergström M, Bockgård N, Dershowitz W, Dessirier B, Frampton A, Fransson Å, Gens A, Gylling B, Hančilová I, Holton D, Jarsjö J, Kim J-S, Kröhn K-P, Malmberg D, Pulkkanen V-M, Sawada A, Sjöland A, Svensson U, Vidstrand P, Viswanathan H, 2018. Conceptual uncertainties in modelling the interaction between engi-neered and natural barriers of nuclear waste repositories in crystalline rock, In: Norris, S., E.A.C. Neeft, and M. Van Geet (eds.): Multiple Roles of Clays in Radioactive Waste Confinement, Geological Society, London, Special Publications, 482, doi: 10.1144/SP482.12, 2018.
Finsterle S, 2019. Overall evaluation of Tasks 8A–D, F, and water-uptake test related to modelling of the interaction between engineered and natural barriers. Task 8 of SKB Task Forces EBS and GWFTS. SKB TR-17-08, Svensk Kärnbränslehantering AB.
Fransson Å, Åkesson M, Andersson L, 2017. Bentonite Rock Interaction Experiment. Characterisation of rock and installation, hydration and dismantling of bentonite parcels. SKB R-14-11, Svensk Kärnbränslehantering AB.
Task 9 - Modelling REPRO, and LTDE-SD
Task 9 focused on modelling of coupled matrix diffusion and sorption in heterogeneous crystalline rock matrix at depth. This was done in the context of inverse and predictive modelling of tracer concentrations of the in-situ experiments performed within LTDE-SD at the Äspö Hard Rock Laboratory in Sweden, and the REPRO project at the ONKALO underground rock characterisation facility in Finland, focusing on sorption and diffusion. The ultimate aim was to develop models that in a more realistic way represent retardation in the natural rock matrix at depth.
Background
The topic of radionuclide transport and retardation in the geosphere is of high importance for assessing the safety of geological repositories for radioactive waste in fractured crystalline rock. Matrix diffusion of solutes in the microporous system of the rock matrix is perceived to be well understood and has been discussed for over 60 years. For the past 35 years, matrix diffusion coupled with sorption has been discussed in the context of retardation of radionuclides potentially migrating from a geological repository for nuclear waste. In recent safety assessments, carried out by SKB and Posiva, the importance of solute exchange between flowing fracture water and stagnant pore water has been stressed in regard to the waste containers’ capability of isolating spent nuclear fuel over long time periods.
The experiments
LTDE-SD was an in-situ study that was carried out at Äspö HRL and it focused on tracer transport in the stagnant pore water of the rock matrix. In the experiment, a cocktail of both sorbing and non-sorbing tracers was allowed to contact a natural fracture surface, as well as the unaltered rock matrix, for a time period of 200 days. The experiment was carried out at a depth of about 410 m below sea level.
The REPRO project was in part carried out in-situ in ONKALO at Olkiluoto in Finland, and in part as an extensive laboratory programme. Concerning in-situ experiments; two water phase diffusion and sorption campaigns were carried out from the REPRO niche at about 400 m depth, from which a number of drillholes has been drilled. These campaigns were the WPDE (Water Phase Diffusion Experiment) series of experiments and the TDE experiment (Through Diffusion Experiment). The in-situ part of REPRO aimed to tackle the topics of diffusion, sorption; anion exclusion; and rock matrix anisotropy. In addition, the laboratory part focused on small scale rock characterisation.
Modelling in Task 9
Task 9 was initiated in the spring of 2015 and started with semi-predictive modelling of the REPRO project. The second subtask focuses on the inverse modelling of experimental results from the in-situ tracer test LTDE-SD. In a following subtask the penetration profile of Cl-36, Cs-137 and Ni-63 into the LTDE-SD rock matrix will be modelled in a predictive fashion. In addition, the TDE (Through Diffusion Experiment) of REPRO was modelled as well.
As an optional subtask, the increased realism in the solute transport codes was put into the perspective of safety assessment time scales. This was to highlight if different aspects of matrix diffusion and sorption, utilising codes with increased complexity and realism, may have any consequence for long-term retardation.
Task 10 - Validation approaches for models with discrete features
Task 10 – Validation approaches for groundwater flow and transport modelling with discrete features
Among the objectives of the Task Force GWFTS are to develop, test and improve tools for conceptual understanding and simulating groundwater flow and transport of solutes in fractured rocks. The ongoing Task 10 focuses on validation of such models. Several of the participating organizations have realized and experienced that there is a need for validation and confidence building in models addressing flow and transport in discrete features. To address this topic, a pragmatic approach is chosen where predictive modeling is an important tool, which is built on:
- Pragmatic validation consistent with the IAEA definition of “fit for purpose” validation considering the limited spatial and temporal scale of available characterization data.
- Use of multiple conceptual and numerical models to quantify uncertainties/sensitivities due to underlying concepts, parameters and assumptions.
- Confidence building considering model conditioning, calibration and rejection. Conditioning and calibration data and methods may be particularly important where site-specific predictions are based on limited/sparse data.
- Sensitivity and uncertainty assessment of key parameters.
- Progressive validation as additional data is collected, guided by the results of previous data limitations and model studies.
- Robust model audit to identify and evaluate assumptions and limitations to ensure transparency.
- Prediction-outcome exercises to evaluate whether a model is an adequate representation of the real system being modelled by comparing model predictions with in-situ and laboratory observations.
Initially, a White Paper was written to guide the modelling work in Task 10. The White Paper (Lanyon et al. 2021) is, among other things, setting out a preliminary view on a pragmatic validation approach. In the first validation exercises, data from granite rock blocks extracted from the Flivik quarry, in the vicinity of Äspö HRL, are used. The objectives of these exercises are:
- Development of concepts and models for flow and transport at the single fracture scale.
- Consideration of the importance of hydro-mechanical coupling (normal loading only) on flow and transport.
- Development of modelling approaches for prediction of:
- Flow and transport in single fractures.
- Upscaled fracture properties from borehole to deposition hole scale.
- Building a starting point for pragmatic validation, i.e., a prediction-outcome exercise.
In the coming sub-tasks, the same type of topics will be addressed, but for larger scales and models including networks of discrete features. In the next coming sub-task, validation of models for flow and transport in the block-scale will tentatively be dealt with. As a final exercise of Task 10, validation of models in the repository tunnel-scale will be addressed according to the plan. At this scale, the models will likely contain a tunnel and a few deposition holes.
Present outline of Task 10
Sub-task | Main activity/modelling topic | Status |
10.1 | Initial White Paper (Lanyon et al. 2021) | Done |
10.2 | Flow and transport in a single fracture | Ongoing |
10.3 | Flow and transport in small to moderate sized models (block-scale) with networks of discrete features | Planned |
10.4 | Flow and transport in larger sized models (repository tunnel-scale) with networks of discrete features | Planned |
References
Lanyon, G. W., Davy, P., Dershowitz, W. S., Finsterle, S., Gylling, B., Hyman, J. D., Neretnieks, I., and M. Uchida, 2021. Pragmatic Validation Approach for Geomechanics, Flow, and Transport Models in Fractured Rock Masses. Paper (DFNE 21-2369) presented at the 3rd International Discrete Fracture Network Engineering Conference, Virtual, June 2021.